Communications using quantum computation
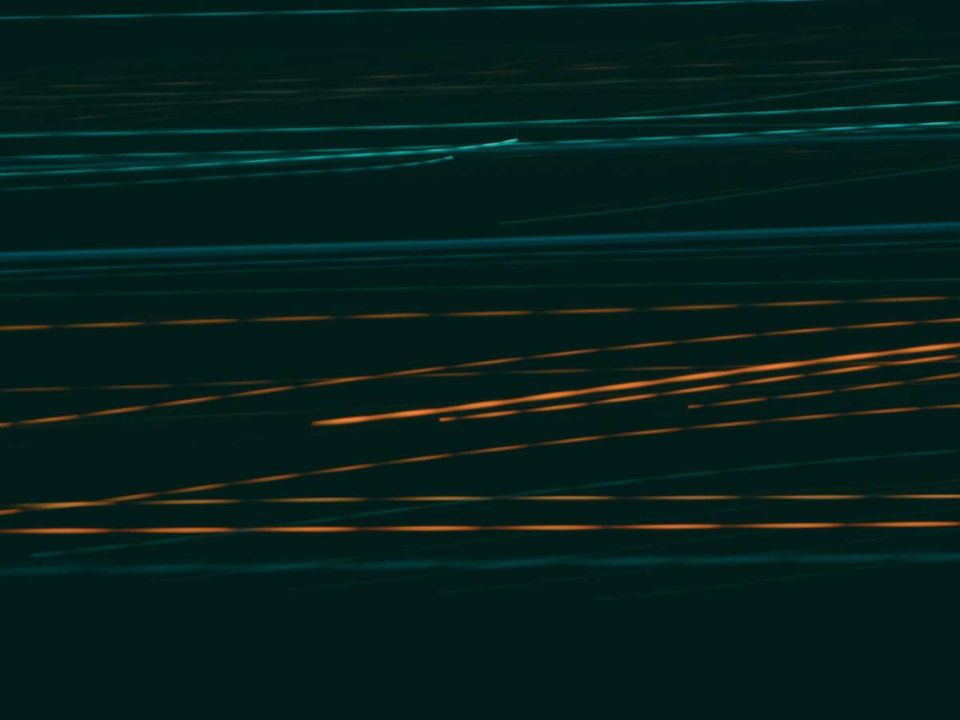
Quantum communication is a field of quantum information science that aims to securely transmit information using the principles of quantum mechanics. In this article, we will discuss the technical aspects of quantum communication and explore how it works.
Quantum Communication
Classical communication relies on the transmission of bits of information, which are represented by either a 0 or a 1. In contrast, quantum communication relies on the transmission of qubits, which are quantum bits. A qubit can be in a superposition of both 0 and 1 states, which means that it can represent both values simultaneously. This property of qubits is what makes quantum communication so powerful.
There are several different methods of quantum communication, but one of the most well-known is called quantum key distribution (QKD). QKD is a technique for securely sharing cryptographic keys between two parties. The basic idea behind QKD is to use the principles of quantum mechanics to detect eavesdropping attempts.
The key idea behind QKD is that it is possible to transmit information in a way that is secure against eavesdropping, as long as the eavesdropper does not have access to the quantum channel used to transmit the qubits. In other words, if an eavesdropper attempts to intercept the qubits being sent from one party to another, they will necessarily change the state of those qubits, which can be detected by the receiver.
QKD Protocol
The QKD protocol consists of four main steps:
- Key generation: The sender (Alice) generates a sequence of qubits and sends them to the receiver (Bob) over a quantum channel. The qubits are randomly polarized in one of two possible bases (e.g., the horizontal-vertical basis or the diagonal basis).
- Basis selection: After receiving the qubits, Bob randomly chooses one of two possible bases in which to measure each qubit. He records which basis he used for each qubit but does not yet reveal this information to Alice.
- Measurement: Bob measures each qubit using the basis he has selected. After the measurement is complete, he sends a message to Alice, indicating which qubits were measured in which basis.
- Key distillation: Alice and Bob compare the bases they used for each qubit. If they used the same basis, they keep the corresponding bit as part of their shared secret key. If they used different bases, they discard the corresponding bit.
The security of the QKD protocol depends on the fact that an eavesdropper (Eve) cannot intercept the qubits without being detected. If Eve tries to intercept the qubits, she will necessarily change the polarization of the qubits, which will be detected by Bob during the measurement phase. This detection allows Alice and Bob to discard any qubits that were intercepted and to continue with the protocol using only the qubits that were not intercepted.
Types of QKD
There are two main types of QKD: prepare-and-measure QKD and entanglement-based QKD.
Prepare-and-measure QKD is the simplest and most commonly used type of QKD. In this method, Alice prepares a sequence of qubits and sends them to Bob over a quantum channel. Bob measures each qubit and sends the results back to Alice. Alice and Bob then use the results of the measurements to distill a shared secret key.
Entanglement-based QKD, on the other hand, relies on the use of entangled qubits. In this method, Alice and Bob share a pair of entangled qubits, which they use to generate a shared secret key. The advantage of entanglement-based QKD is that it is less vulnerable to certain types of attacks, such as photon number splitting attacks, which can be used to compromise prepare-and-measure QKD protocols.
Challenges in Quantum Communication
One of the biggest challenges in quantum communication is the issue of channel loss and noise. In any communication channel, there is a certain amount of noise and loss that occurs as the signal is transmitted. In classical communication, this can be overcome by using error-correcting codes. However, in quantum communication, the noise and loss of the qubits cannot be corrected using classical error-correcting codes because they violate the no-cloning theorem of quantum mechanics.
Instead, researchers have developed a number of strategies to deal with noise and loss in quantum communication. One such strategy is to use quantum repeaters, which are devices that can extend the range of a quantum channel by repeating the qubits. Another strategy is to use quantum error-correcting codes, which allow errors to be detected and corrected without violating the no-cloning theorem.
Another challenge in quantum communication is the issue of scalability. Most quantum communication systems today are limited to a few hundred kilometers of transmission range, which is far less than the range of classical communication systems. This is partly due to the limitations of current technology, but it is also a fundamental challenge of quantum communication.
To overcome this challenge, researchers are exploring the use of quantum networks, which are composed of many interconnected quantum devices. In a quantum network, information can be transmitted over multiple paths, which can increase the range and reliability of the communication. However, building a large-scale quantum network presents many technical and theoretical challenges.
Applications of Quantum Communication
Quantum communication has a number of potential applications in areas such as cryptography, telecommunications, and computing.
One of the most promising applications of quantum communication is in quantum cryptography, which uses the principles of quantum mechanics to provide secure communication. Quantum cryptography offers several advantages over classical cryptography, including resistance to eavesdropping attacks and the ability to detect when a communication has been intercepted.
Another application of quantum communication is in quantum teleportation, which is a technique for transmitting quantum information between two distant locations without physically transmitting the qubits. Quantum teleportation has the potential to revolutionize areas such as quantum computing and quantum sensing.
Finally, quantum communication could be used to improve the speed and efficiency of quantum computing. One of the challenges of quantum computing is the issue of entanglement generation and measurement, which requires large amounts of computational resources. By using quantum communication to distribute entangled qubits, the resources required for entanglement generation and measurement could be greatly reduced.
Conclusion
Quantum communication is a promising field of quantum information science that has the potential to revolutionize communication, cryptography, and computing. While there are still many technical and theoretical challenges to be overcome, researchers are making rapid progress in developing new techniques and technologies for quantum communication. As these technologies mature, we can expect to see quantum communication playing an increasingly important role in our lives.